logarithmic%20integral
(0.003 seconds)
11—20 of 26 matching pages
11: 22.3 Graphics
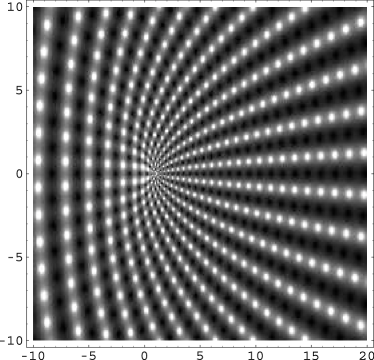
12: 7.23 Tables
Abramowitz and Stegun (1964, Chapter 7) includes , , , 10D; , , 8S; , , 7D; , , , 6S; , , 10D; , , 9D; , , , 7D; , , , , 15D.
Abramowitz and Stegun (1964, Table 27.6) includes the Goodwin–Staton integral , , 4D; also , , 4D.
Zhang and Jin (1996, pp. 637, 639) includes , , , 8D; , , , 8D.
Zhang and Jin (1996, pp. 638, 640–641) includes the real and imaginary parts of , , , 7D and 8D, respectively; the real and imaginary parts of , , , 8D, together with the corresponding modulus and phase to 8D and 6D (degrees), respectively.
Zhang and Jin (1996, p. 642) includes the first 10 zeros of , 9D; the first 25 distinct zeros of and , 8S.
13: 10.75 Tables
The main tables in Abramowitz and Stegun (1964, Chapter 9) give to 15D, , , , to 10D, to 8D, ; , , , 8D; , , , , 5D or 5S; , , , , 10S; modulus and phase functions , , , , 8D.
Zhang and Jin (1996, p. 270) tabulates , , , , , 8D.
Bickley et al. (1952) tabulates or , or , , (.01 or .1) 10(.1) 20, 8S; , , , or , 10S.
The main tables in Abramowitz and Stegun (1964, Chapter 9) give , , , , 8D–10D or 10S; , , , ; , , , 8D; , , , , 5S; , , , , 9–10S.
Zhang and Jin (1996, p. 271) tabulates , , , , , 8D.
14: 3.4 Differentiation
15: 12.10 Uniform Asymptotic Expansions for Large Parameter
16: Bibliography K
17: 11.6 Asymptotic Expansions
18: Bibliography
19: 9.18 Tables
Miller (1946) tabulates , for , for ; , for ; , for ; , , , (respectively , , , ) for . Precision is generally 8D; slightly less for some of the auxiliary functions. Extracts from these tables are included in Abramowitz and Stegun (1964, Chapter 10), together with some auxiliary functions for large arguments.
Zhang and Jin (1996, p. 337) tabulates , , , for to 8S and for to 9D.
Sherry (1959) tabulates , , , , ; 20S.